On 25 October 2010 at 9:42 PM local time, the people living on the southern Mentawai islands, a chain of islands located off Sumatra, Indonesia, felt a slow, gentle rocking of the rocks below their feet1. Initial reports of the earthquake indicated that a magnitude-7 to -7.2 earthquake had occurred seaward of the islands2. Five to 10 minutes later, a large wave inundated the western coasts of the islands, locally flooding areas more than 10 metres above the ground surface1. Together, the earthquake and tsunami killed more than 400 people and left 300 missing.
Photos showing tsunami damage on Sibigau Island. (a) shows an aerial view, and (b), a ground-based view from the forest trimline (Source: Hill et al, 2012)
Such a large tsunami would typically require an earthquake of magnitude greater than 8. In this case, while the early data from the Mentawai earthquake suggested a much smaller event, a more complete assessment of the magnitude determined that it was magnitude-7.8 — roughly two thirds as energetic as a magnitude-8.
This kind of earthquake, where the tsunami was bigger than expected based on the ground shaking, falls into a special class of events called ‘tsunami earthquakes’. These earthquakes display distinct characteristics compared to most earthquakes: they rupture more slowly, take longer than usual, and occur close to the trench of the subduction zone (figure 1) — the place where the plate boundary reaches the surface2, 3.
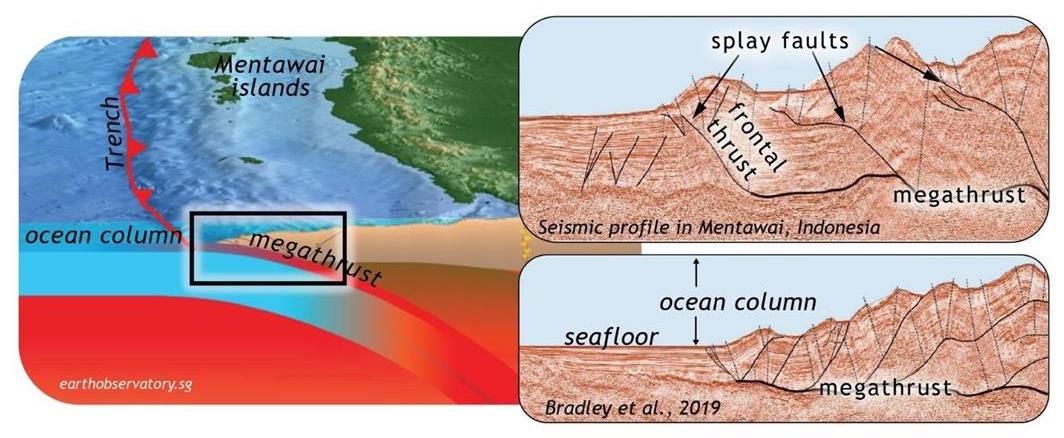
Figure 1: On the left is a schematic diagram of the Sumatran subduction zone. Tsunami earthquakes occur at the shallow part of the subduction zone (indicated by a black rectangle), which is close to the trench. Note that the ocean becomes deeper closer to the trench. The right shows interpreted seismic profiles acquired in the shallow part of the subduction zone. The steeply dipping splay faults sole to the gently dipping megathrust. The splay fault nearest to the trench is the frontal thrust. The water depth above the frontal thrust is deeper compared to that above the splay faults farther from the trench (Sources: Earth Observatory of Singapore (left image) and Bradley et al., 2019 (seismic profiles on the right))
Tsunami earthquakes have been detected along most major subduction zones. They pose a major hazard because there typically isn’t time to understand the earthquake well enough to send out a tsunami warning. If we can understand the physics of how they form, we may be able to tell which subduction zones can produce them and which can’t, and plan accordingly. But scientists are still trying to figure out why these kinds of earthquakes are so effective at generating large tsunamis.
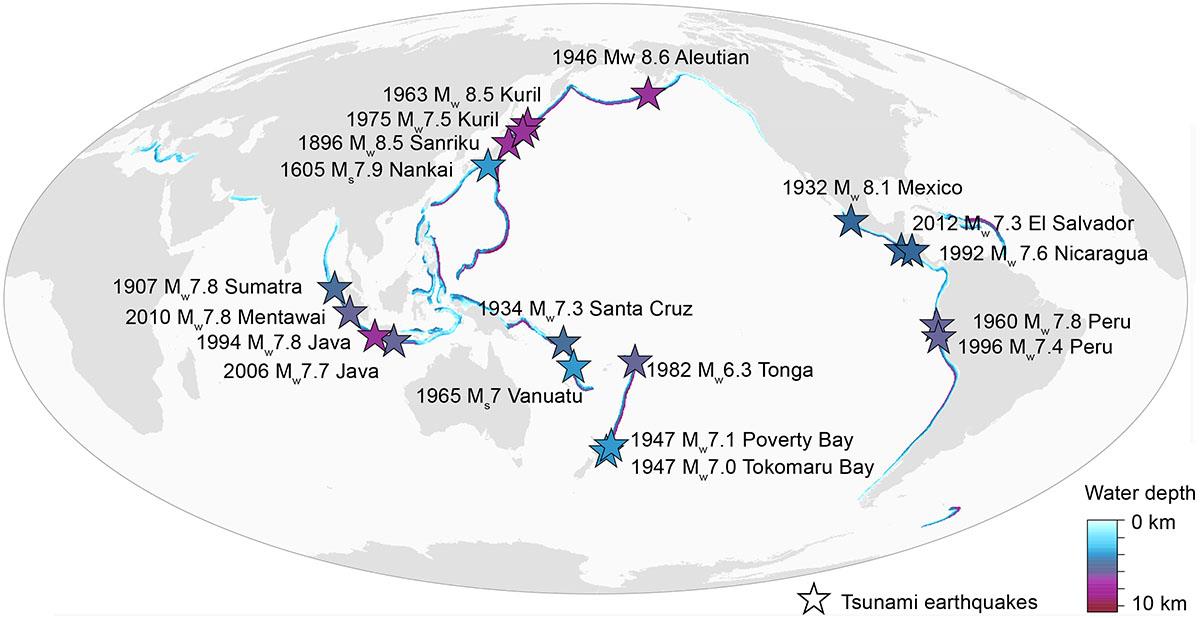
Figure 2: Recorded tsunami earthquakes and associated water depths at subduction zones (Source: Felix et al., 2021)
In our study published in the Bulletin of the Seismological Society of America, we decided to test one particular hypothesis: that tsunami earthquakes are caused by slip on splay faults, i.e., steep faults that break up from the primary plate-boundary fault (the megathrust), which is close to horizontal near the trench (figure 1). Several studies have proposed that this mechanism should be a way to efficiently lift the ocean column because slip on a steep fault will lift the ocean more than slip on a gently dipping fault4. We focused first on splay faults located at the trench, and then expanded our study to include splays located anywhere in the shallow part of the subduction zone (figure 1).
What we found was surprising. By looking at a variety of scenarios for how the crust can deform (figure 3), we determined that splay faults do indeed generate more potential tsunami energy than equivalent slip on the plate-boundary fault. However, because the ocean is so deep and the uplift patches of the splay faults are long but narrow (elongated), the vast majority of this energy from the splay faults will be dissipated into the ocean rather than transmitted to the ocean surface as a tsunami (blue curved lines in figure 3). In other words, a narrow zone of high uplift on the seafloor will still only produce a broad, gentle wave, almost identical to a wave generated without a splay fault. This effect is more significant for deep ocean columns and narrow uplift patches.
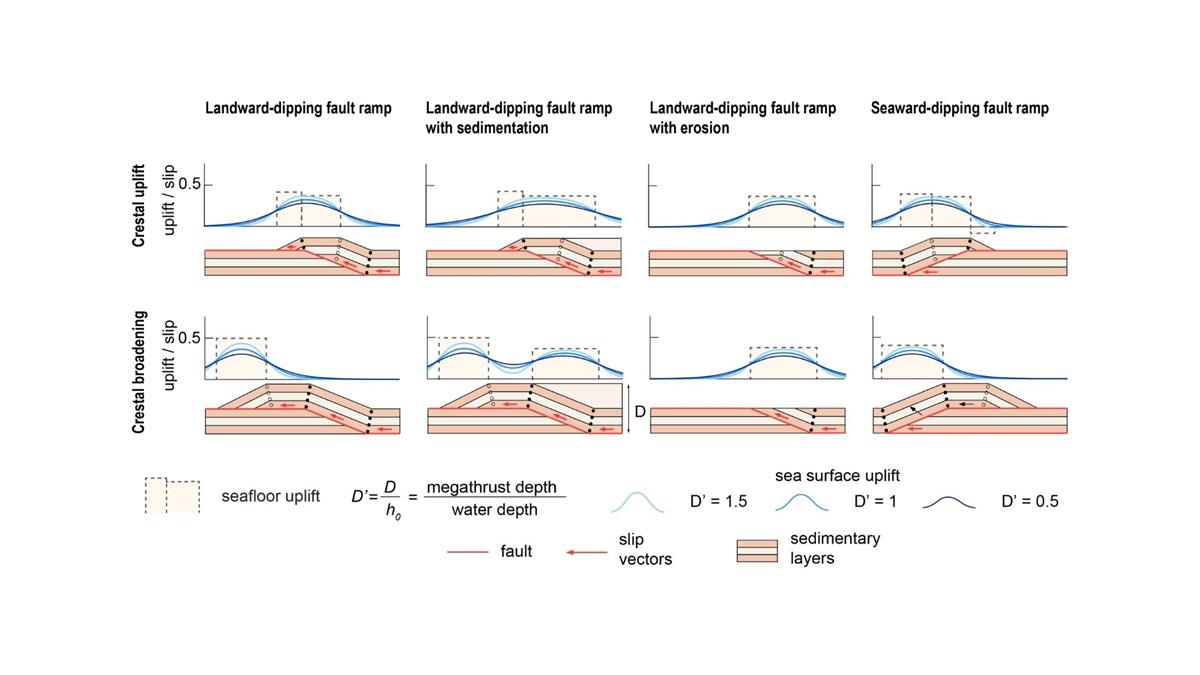
Figure 3: Structural models of frontal thrust ramps with associated seafloor and sea surface uplift patterns. Dashed boxes show vertical deformation of the seafloor relative to the downgoing plate. Curved lines are the resulting sea surface deformation for different ratios of the megathrust depth to the water depth. Increasing D’ widens the seafloor uplift patch relative to the water depth, and therefore decreases the effect of water damping (lighter blue curves represent less water damping) (Source: Felix, et al., 2021)
While tsunami scientists have known about this dissipation effect for decades5, the application of this knowledge to the geometry of real subduction zones has been missing. We, therefore, mapped out a set of subduction zones that have experienced tsunami earthquakes and showed that for these regions, the frontal thrust located at the tip of the subduction zone will have a minor impact. For the Mentawai earthquake, in particular, the frontal thrust contributed less than 10 per cent of the total energy in the tsunami (figure 4).
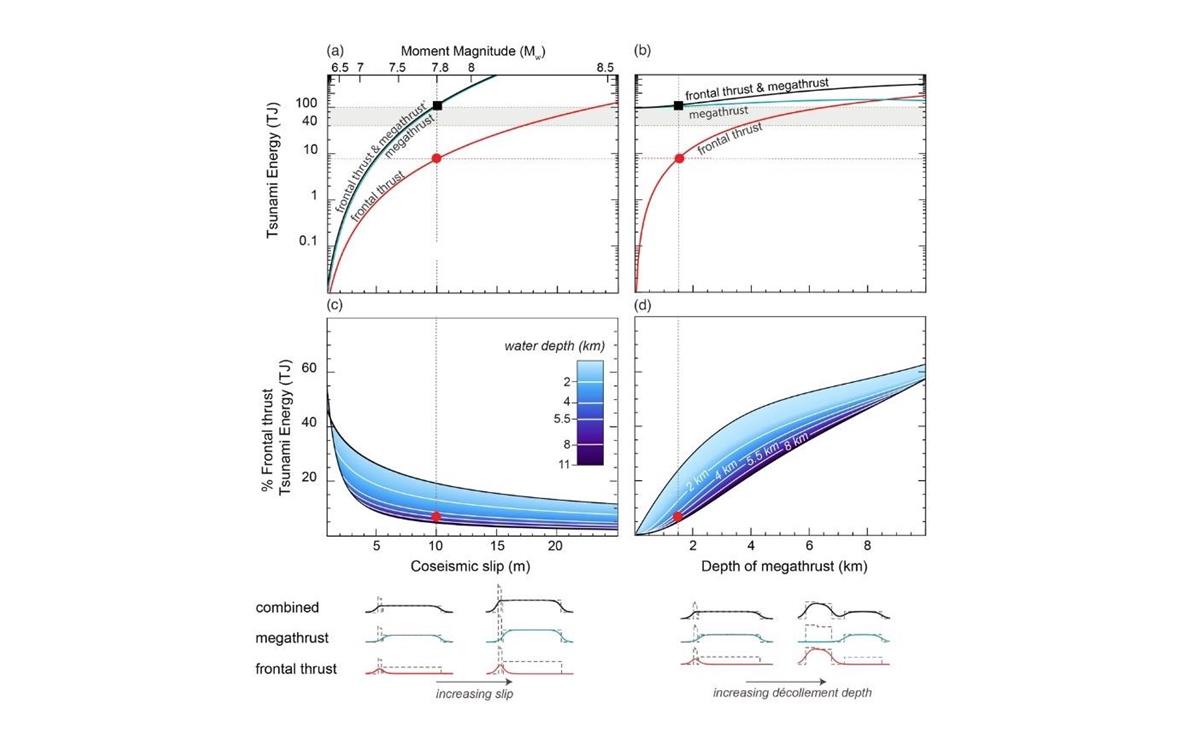
Figure 4: Tsunami energy contributed by the frontal thrust compared to the megathrust for the Mentawai tsunami earthquake. (a) Varying coseismic slip and (b) varying the depth of the megathrust. The circles and squares correspond energies for the expected Mentawai event. (c and d) The percentage of total tsunami energy contributed by the frontal thrust for different water depths. The frontal thrust at 5.5 kilometres water depth (red circle, equivalent to the Mentawai earthquake) has minimal contribution to the overall tsunami energy (Source: Felix, et al., 2021)
Splay faults can still be important, however, if they are located landward of the trench. This is because the plate-boundary fault deepens landward so that splay faults will lift up wider and wider patches. The ocean also shallows landward. Together, these effects mean that more and more of the potential tsunami energy will be transmitted to the surface for splay faults connected to the deeper part of the plate-boundary fault (figure 4).
For the 2010 Mentawai earthquake, this still leaves an open question: what caused the tsunami to be so large? Various studies have tried to answer this question, to varying levels of success. From our work, we know that large tsunamis must be sourced by broad regions of uplift above the wider plate boundary fault (megathrust). However, while earthquakes on megathrusts like the Sunda megathrust in Indonesia and the Aleutian megathrust offshore Alaska are relatively common, they only occasionally trigger large tsunamis. More observations and more studies of past and future tsunami earthquakes will be needed to answer this question.
References
1 Hill, Emma M., Jose C. Borrero, Zhenhua Huang, Qiang Qiu, Paramesh Banerjee, Danny H. Natawidjaja, Pedro Elosegui et al. "The 2010 Mw 7.8 Mentawai earthquake: Very shallow source of a rare tsunami earthquake determined from tsunami field survey and near‐field GPS data." Journal of Geophysical Research: Solid Earth 117, no. B6 (2012)
2 Newman, Andrew V., Gavin Hayes, Yong Wei, and Jaime Convers. "The 25 October 2010 Mentawai tsunami earthquake, from real‐time discriminants, finite‐fault rupture, and tsunami excitation." Geophysical Research Letters 38, no. 5 (2011).
3 Kanamori, Hiroo. "Mechanism of tsunami earthquakes." Physics of the earth and planetary interiors 6, no. 5 (1972): 346-359.
4 Satake, K., and Y. Tanioka. "Sources of tsunami and tsunamigenic earthquakes in subduction zones." Pure and Applied Geophysics154, no. 3 (1999): 467-483.
5 Kajiura, Kinjiro. "The leading wave of a tsunami." 東京大學地震研究所彙報= Bulletin of the Earthquake Research Institute, University of Tokyo 41, no. 3 (1963): 535-571.
Additional reference
Bradley, Kyle, Yanfang Qin, Helene Carton, Nugroho Hananto, Fernando Villanueva‐Robles, Frederique Leclerc, Wei Shengji, Paul Tapponier, Kerry Sieh, and Satish Singh. "Stratigraphic control of frontal décollement level and structural vergence and implications for tsunamigenic earthquake hazard in Sumatra, Indonesia." Geochemistry, Geophysics, Geosystems 20, no. 3 (2019): 1646-1664