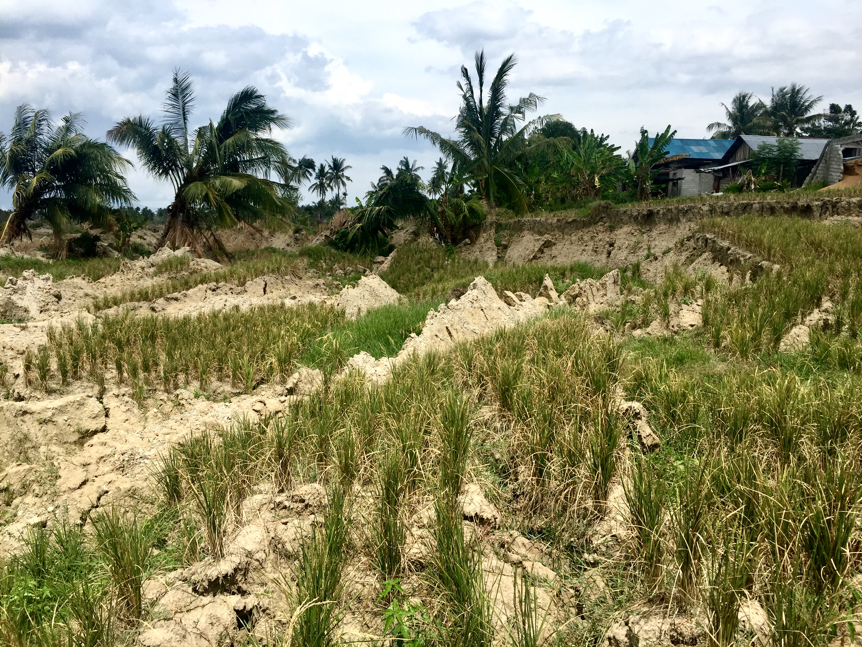
During the early evening of 28 September 2018, a magnitude-7.5 earthquake struck along the coast of northwestern Sulawesi, Indonesia. This region hosts a famous strike-slip fault system called the Palu-Koro Fault.
Even though this is one of the fastest slipping faults in the world, it has not produced many large earthquakes during historical times. The Palu-Koro Fault was therefore thought to have a high probability of a large and destructive earthquake, and so the occurrence of the 2018 quake in Sulawesi wasn’t very surprising.
However, the landsliding that was triggered by the earthquake turned out to be unexpectedly destructive. Soon after the earthquake, witnesses described entire villages south of Palu City sinking into mud and disappearing, and it was apparent that ground liquefaction was at least partially to blame for the landsliding.
Liquefaction occurs when sandy deposits below ground, which are saturated with ground water, are struck by strong seismic waves. Jostled by the ground shaking, the sand tries to compact. If only air filled the pore space between the sand grains, which is common in places with a low water table, this compaction would occur rapidly and would not be detectable at the surface. However, because water is incompressible, in saturated layers the sudden compaction causes a rapid spike in water pressure. This pressure effectively ‘floats’ the sand grains, which reduces and eventually overcomes the friction that holds the sediment together. The sand layer suddenly transforms from a soft rock into a thick fluid. Some of the highly pressurised water and sand can escape from this fluidised zone and erupt to the surface, sometimes jetting up to 20 metres into the air.
The main danger of liquefaction is that ground slopes which were previously stable can suddenly become detached from the buried sediments beneath them, with the effectively frictionless fluid layer forming a lubricated surface for sliding. The still relatively solid ground above the liquid layer breaks up as it slides down the slope, forming what is called a lateral spread. Two famous examples of lateral spreading occurred in 1964, when separate great earthquakes struck Japan and Alaska. Lateral spreading has typically damaged cities on coasts or along large low-elevation rivers, and has been viewed as a mainly economic hazard.
Lateral spreading in the Palu Valley was surprising because it occurred on relatively steep slopes far away from the coast or a major river channel. The affected area had a slope of about 1°, which doesn’t sound very steep but is actually much steeper than previously documented lateral spreads, which occurred on slopes between 0° and 0.5°.
Landsliding in the eastern Palu Valley also happened on the surface of alluvial fans, which are geological features that formed over time by streams carrying coarse sediment from the nearby mountain range. Such large-scale liquefaction and landsliding had not previously been observed on alluvial fans. The main questions that emerged for the Palu landslides was why this type of landsliding happened in such an unusual setting.
To answer this, we studied Google EarthTM and PlanetTM satellite images taken before and after the Palu earthquake, and eventually observed a striking pattern in the location of the landsliding. We noticed that a large irrigation aqueduct clearly separated a dry and mostly uncultivated region above the aqueduct from a lush and densely cultivated area below, dominated by rice paddies. This area just below the aqueduct is where lateral spreading occurred, while dry areas above were unaffected. We also observed that the lower edge of the landslides was located where rice paddies at higher elevation changed into mixed paddies and coconut palm groves at lower elevation.
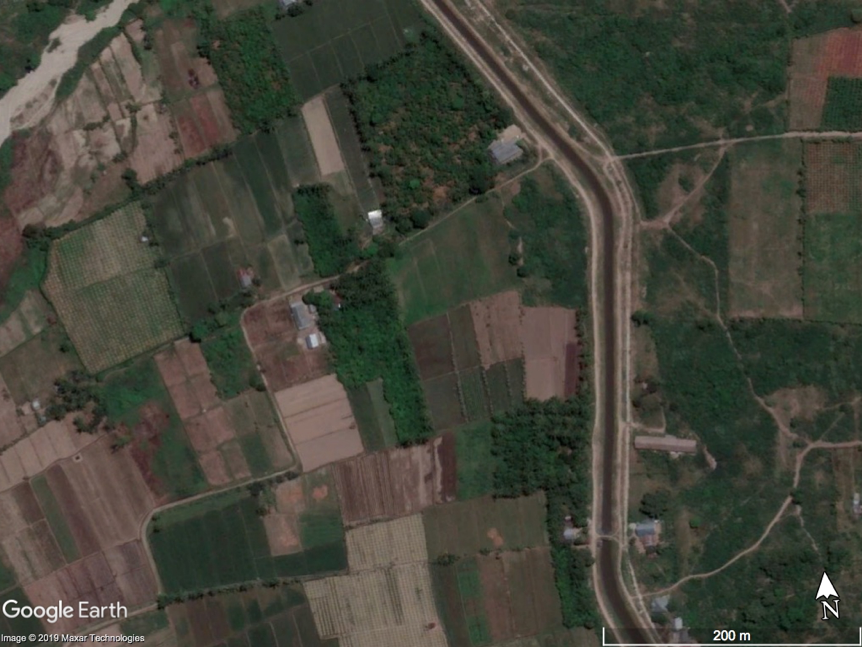
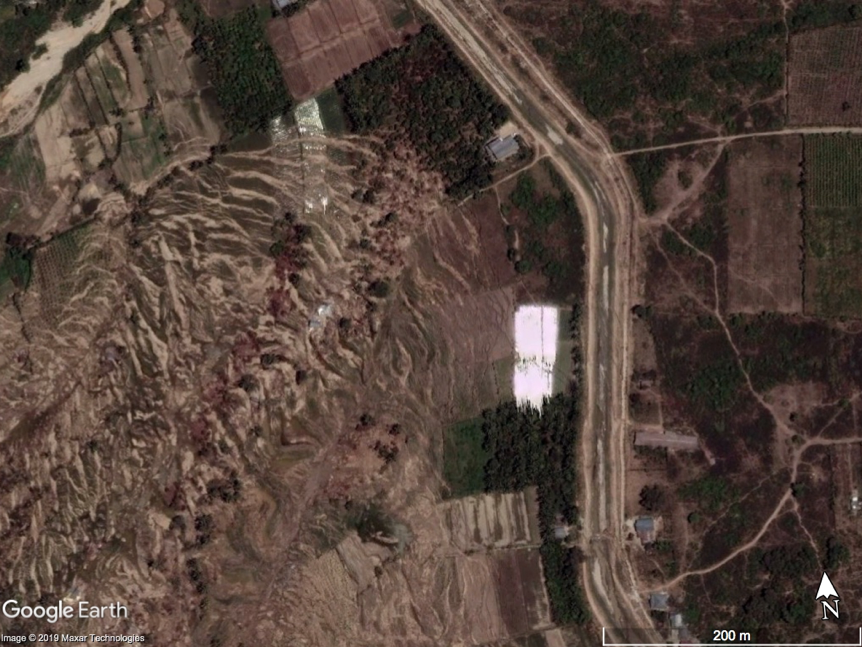
We hypothesised that the water that was being drawn from (or slowly leaking from) the aqueduct had significantly raised the water table in the heavily irrigated areas, saturating sandy layers beneath the surface. This created a new liquefaction hazard that was then triggered by the earthquake. Areas that were not irrigated (above the aqueduct) or were less heavily irrigated (forested land) were not affected by liquefaction because the water table was closer to its natural level, where the sediments are already somewhat compacted by the weight of the younger sediments on top and liquefaction is not possible.
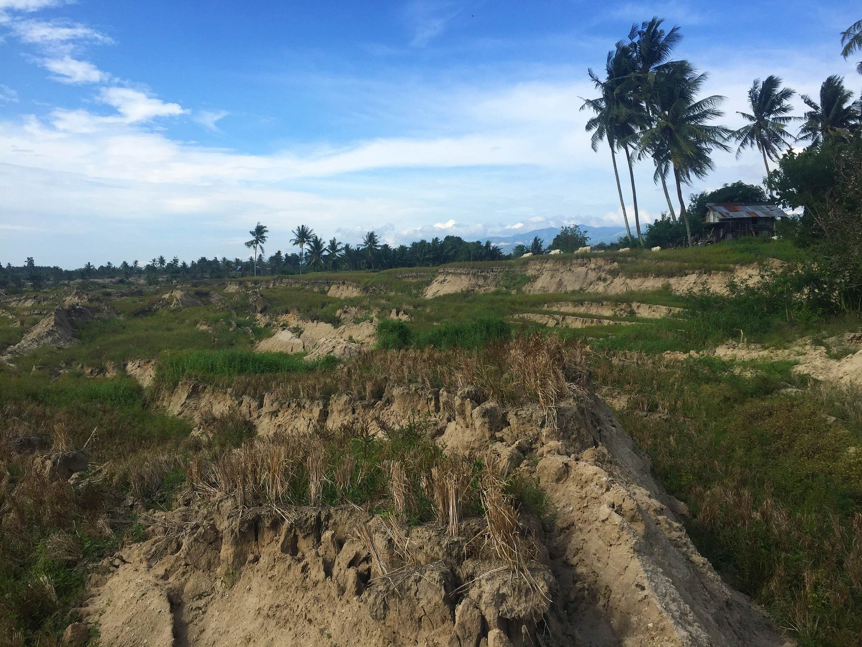
The greatest loss of life due to landsliding can certainly be attributed to four major landslides, which happened where the aqueduct crossed slightly steeper slopes (>1.5°). In these areas, the ground that detached was able to gather enough momentum to travel great distance downslope, leaving behind a featureless region of broken ground. We were able to identify individual structures that moved more than 750 meters down slope.
As part of the study, an international team of scientists led by researchers from the Tsunami and Disaster Mitigation Research Center (TDMRC) at Syiah Kuala University took a firsthand look at these landslide areas several months after the earthquake. They saw evidence for liquefaction and the broken remnants of rice paddies just below the aqueduct.
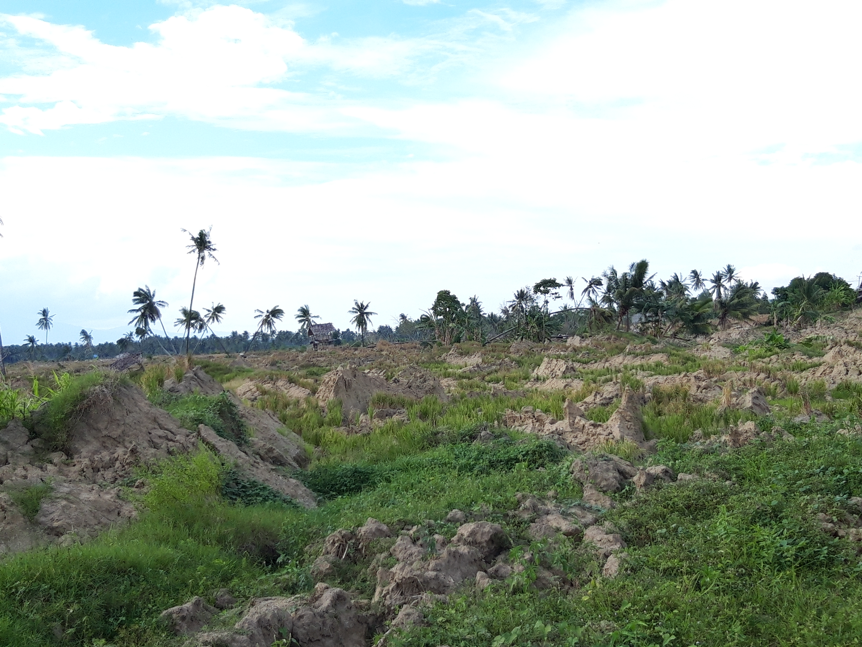
What is the future of hazard in the Palu Valley? Agriculture is a central part of the economy and the irrigation of the Palu Valley has been a large economic benefit to the region for more than a century. However, it is now clear that this irrigation is also dangerous due to the continuing potential for large earthquakes and liquefaction. Our observations suggest that more proactive management of the water table by intermixed planting of rice with crops that need less water could help increase resilience to liquefaction landsliding.
The Palu Valley is not the only place where irrigation has raised the water table in the vicinity of an active fault. The disaster at Palu should be used as a motivation for discovering other dangerous areas that have not yet been tested by a large earthquake.
Here are the findings from Dr Kyle Bradley's paper that was published in Nature Geoscience on 27 Sepetember 2019.
(Source of thumbnail image: Devina Andiviaty/Wikimedia Commons)