Volcanic eruptions are sometimes accompanied by clouds of ash and hot gases that travel down the slopes of volcanoes at tens of kilometres per hour. These clouds, called pyroclastic density currents (PDCs), are some of the deadliest volcanic hazards. And as we cannot easily study them in real-time, we took another approach to quantify some of the features that make them so dangerous: their speed, temperature, height, and how far they travelled.
PDCs are often controlled by gravity. They therefore usually flow from high to low elevation and follow the easiest path allowed by topography. This means that PDCs most frequently flow in channels, allowing scientists and government planners to foresee which direction they might go in the event of an eruption.
Although they travel mostly in valleys, they can also unexpectedly overflow and affect inhabited areas under certain conditions and become unconfined, for example, when they aren’t nearly as controlled by topography. As a result, they can flow outside of channels and sometimes even up slopes. This is even more dangerous than usual, as these types of PDCs may flow unexpectedly into inhabited areas where they can cause severe damage, injury, and death. When that happens, these clouds are extremely difficult to plan for because we know so little about them.
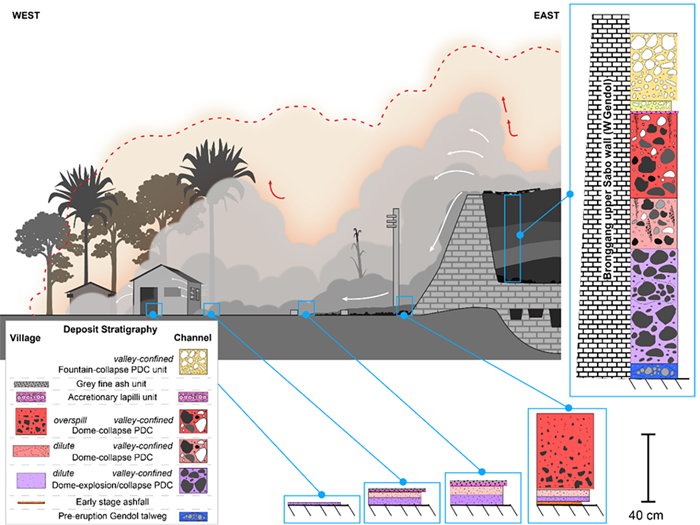
Figure showing how an unconfined PDC can flow out of its channel and into an inhabited area. The coloured columns represent the deposits at different distances from the channel and show that even though far from the channel the PDC and its deposits may be very thin, they can still be extremely deadly (Source: Geoff Lerner and Phuong Nguyen/Earth Observatory of Singapore)
Some well-known eruptions have been accompanied by unconfined PDCs. For example, during the famous 1980 Mount Saint Helens eruption in the United States of America, a directed blast-type eruption was unconfined right from the start and impacted a wide area to the north of the volcano. It flattened and burnt trees and killed over 50 people. During the 1997 eruption at Soufriere Hills volcano in Montserrat, thin clouds of extremely hot ash and gas drifted out of their main channel and into several villages. This led to more than 20 casualties, of which most, if not all, were the result of unconfined PDCs.
In our recently published study, we looked at these types of PDCs from two recent eruptions, the 2010 eruption of Merapi in Indonesia and the 2018 eruption of Fuego in Guatemala. In both cases, PDCs that initially started flowing in channels escaped and unexpectedly inundated villages. At Merapi, dense slow-moving flows entered the village of Bakalan and caused a great deal of building damage, while slow and dilute surges of hot ash entered the village of Bronggang. These ash surges were not strong enough to cause building damage but were hot enough to kill or injure over 50 people. At Fuego, multiple types of slow-moving PDCs entered the village of San Miguel Los Lotes, causing severe building damage and killing hundreds to thousands of people.
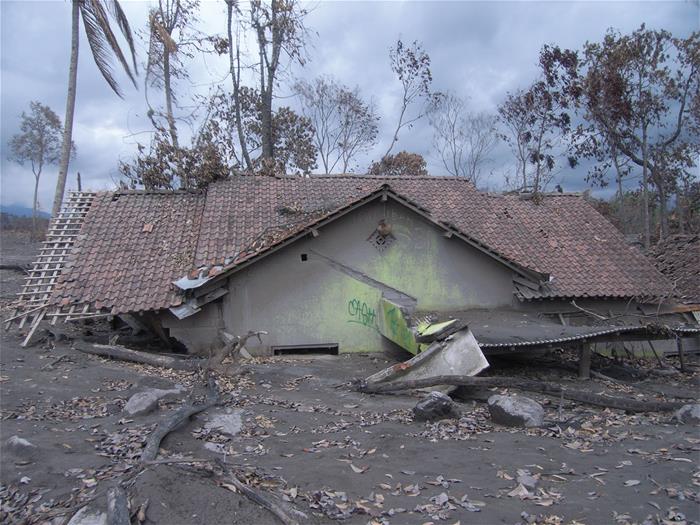
Building damage at Bakalan caused by slow, dense PDCs. We can see that that due to its slow speed, the PDC buried much of the building, but did not completely destroy it (Source: Susanna Jenkins/Earth Observatory of Singapore)
In this study, we wanted to understand some characteristics of these unconfined PDCs as they occur, such as their velocity, temperature, force, height, and how far they travel from the channel. These characteristics are very difficult to measure during an eruption due to the dangerous and unpredictable nature of the PDCs. Instead, we looked at the impacts and deposits from the PDCs from these eruptions after they happened.
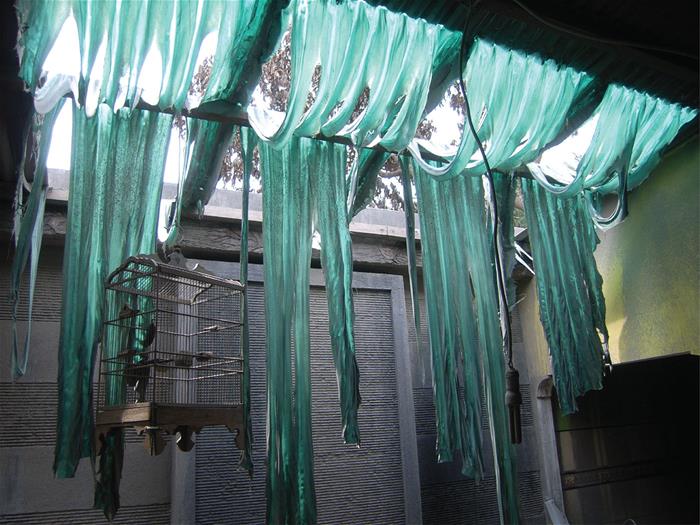
Damage caused by the slow, dilute PDCs at Bronggang. We can see that the PDCs weren’t strong enough to damage the building or knock things over, but were hot enough to severely melt the plastic roof material (>100 °C), which is also hot enough to be deadly to people (Source: Susanna Jenkins/Earth Observatory of Singapore)
At Merapi, some of us were able to assess the impacts and damages in person shortly after the eruption. We could then use the characteristics of the impacts, such as the type and amount of building damage, whether trees were knocked down, the types of injuries, and the characteristics of the deposits, to figure out the dynamics of the PDCs including their temperature, speed, and height.
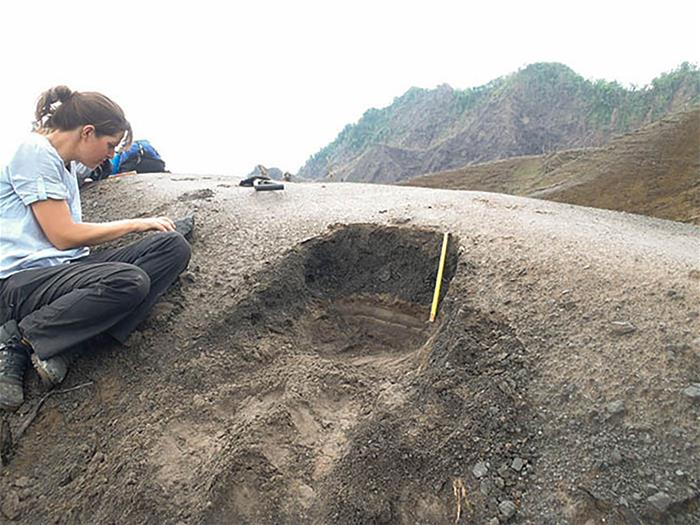
Volcanologist Susanna Jenkins analyses deposits from unconfined pyroclastic density current deposits that were emplaced during the Nov 2010 Merapi eruption (Source: Susanna Jenkins/Earth Observatory of Singapore)
At Fuego, we applied the same principles to study the PDCs remotely. We looked at satellite imagery, aerial photography, and media images to assess impacts and used what we learned from studying Merapi to try to understand the PDC characteristics at Fuego.
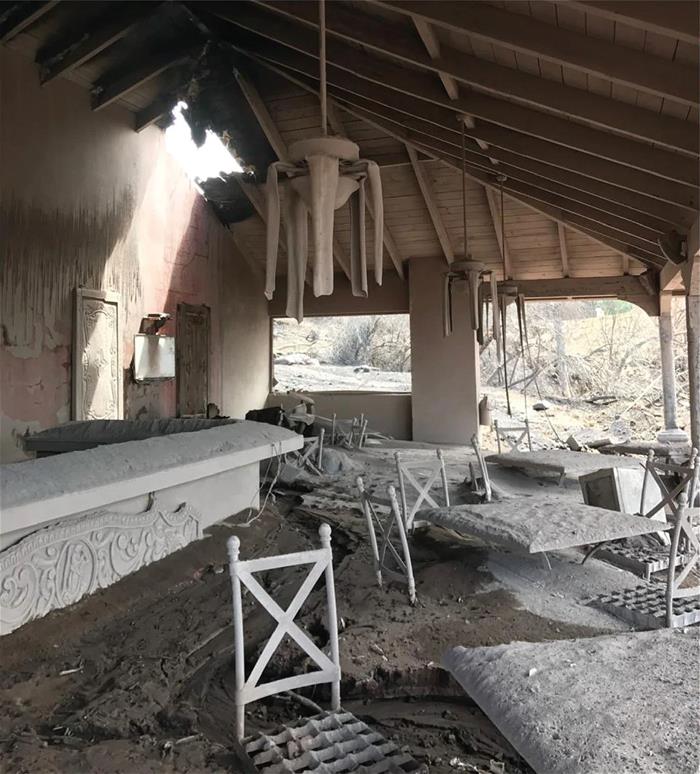
Inside a building damaged by the PDCs in the 2018 Fuego eruption. We can see deposits from the PDC on the table and floor. We can see in the photo that the PDC was a high enough temperature to melt the ceiling fans, but not forceful enough to knock over the chairs or tables (Source: Anonymous)
We also used this study to understand the characteristics of these unconfined PDCs more generally across recorded eruptions, in order to provide useful information to plan for future events. For example, we showed that the types of slow PDCs seen in 2010 at Merapi and 2018 at Fuego only travel at speeds of less than 10 metres per second but can have heights of up to 10 metres and temperatures between 100 to 300 °C. Although slow-moving, these PDCs can still cause severe injury, death, and damage. We also found that PDCs of this type can travel several hundreds of metres from the main channel. This is a characteristic that should be given close attention for future hazard planning at active volcanoes.